Gas sensors have emerged as pivotal devices in various industries, playing a crucial role in monitoring and detecting harmful or flammable gases. With the rapid advancements in technology, gas sensors have evolved significantly, offering enhanced sensitivity, selectivity, and reliability. This article delves into the advancements in gas sensor applications, exploring the latest innovations, technologies, and trends shaping the future of gas detection.

Gas sensors have emerged as pivotal devices in various industries, playing a crucial role in monitoring and detecting harmful or flammable gases. With the rapid advancements in technology, gas sensors have evolved significantly, offering enhanced sensitivity, selectivity, and reliability. This article delves into the advancements in gas sensor applications, exploring the latest innovations, technologies, and trends shaping the future of gas detection.
Historical Evolution of Gas Sensors
The history of gas sensors dates back to the early 19th century, with the development of the first chemical gas detection methods. However, it was not until the mid-20th century that significant progress was made in creating practical and reliable gas sensors. Early gas sensors relied on chemical reactions or changes in electrical conductivity to detect gases. These sensors were often bulky, had limited sensitivity, and required frequent maintenance.
Advancements in materials science, microelectronics, and nanotechnology have revolutionized gas sensors. Modern gas sensors are smaller, more accurate, and capable of detecting a wider range of gases at lower concentrations. The evolution of gas sensors can be broadly categorized into several generations, each characterized by significant technological leaps.
- First-Generation Gas Sensors: These sensors relied on chemical reactions to detect gases. Examples include catalytic bead sensors for detecting combustible gases and metal oxide semiconductor (MOS) sensors for detecting reducing gases.
- Second-Generation Gas Sensors: These sensors utilized electrochemical principles, offering improved sensitivity and selectivity. Electrochemical sensors are widely used for detecting toxic gases such as carbon monoxide (CO) and hydrogen sulfide (H2S).
- Third-Generation Gas Sensors: This generation introduced optical sensors, which utilize the absorption, reflection, or fluorescence of light to detect gases. Optical sensors are highly selective and can operate at high temperatures, making them suitable for harsh environments.
- Fourth-Generation Gas Sensors: The latest generation of gas sensors leverages nanotechnology and advanced materials such as graphene, carbon nanotubes, and metal-organic frameworks (MOFs). These sensors offer unprecedented sensitivity, selectivity, and stability, enabling the detection of trace gases at parts-per-million (ppm) or even parts-per-billion (ppb) levels.
Types of Gas Sensors
Gas sensors can be classified based on their operating principles, detection mechanisms, and target gases. Some of the most common types of gas sensors include:
- Metal Oxide Semiconductor (MOS) Sensors:
- Operating Principle: Changes in electrical conductivity due to gas adsorption on the sensor surface.
- Applications: Detection of reducing gases (e.g., CO, H2, NH3) and oxidizing gases (e.g., NO2, O3).
- Advantages: Low cost, simple manufacturing process, and wide detection range.
- Disadvantages: Limited selectivity and sensitivity to humidity and temperature changes.
- Electrochemical Sensors:
- Operating Principle: Electrochemical reactions between the target gas and an electrolyte, resulting in a measurable current.
- Applications: Detection of toxic gases (e.g., CO, H2S, SO2) and oxygen.
- Advantages: High sensitivity and selectivity, fast response time.
- Disadvantages: Limited lifetime due to electrolyte consumption, and sensitivity to temperature and humidity.
- Optical Sensors:
- Operating Principle: Absorption, reflection, or fluorescence of light by the target gas.
- Applications: Detection of a wide range of gases, including hydrocarbons, CO2, and volatile organic compounds (VOCs).
- Advantages: High selectivity, long lifetime, and operation at high temperatures.
- Disadvantages: High cost and complexity.
- Catalytic Bead Sensors:
- Operating Principle: Catalytic combustion of the target gas on a heated bead, resulting in a temperature change.
- Applications: Detection of combustible gases (e.g., methane, propane).
- Advantages: Simple design and low cost.
- Disadvantages: Limited selectivity and sensitivity to environmental conditions.
- Solid-State Sensors:
- Operating Principle: Changes in electrical properties (e.g., resistance, capacitance) due to gas adsorption.
- Applications: Detection of a variety of gases, including oxygen, nitrogen dioxide, and ammonia.
- Advantages: High stability and reliability, low power consumption.
- Disadvantages: Limited detection range and sensitivity.
Recent Advancements in Gas Sensor Technology
Recent advancements in gas sensor technology have focused on improving sensitivity, selectivity, stability, and reducing the size and cost of sensors. Some of the key developments include:
- Nanotechnology-Based Sensors:
- Graphene-Based Sensors: Graphene, a single layer of carbon atoms, exhibits exceptional electrical and mechanical properties. Graphene-based gas sensors offer high sensitivity and fast response times. Research has shown that graphene can detect gases such as NO2, NH3, and CO at ppm levels.
- Carbon Nanotubes (CNTs): CNTs have a high surface-to-volume ratio, making them ideal for gas sensing. CNT-based sensors can detect a wide range of gases, including VOCs, CO, and H2.
- Metal-Organic Frameworks (MOFs): MOFs are crystalline materials with high porosity and large surface areas. They can be tailored to selectively adsorb specific gases, making them ideal for highly selective gas sensing.
- Smart Gas Sensors:
- Integration with IoT: The integration of gas sensors with the Internet of Things (IoT) enables real-time monitoring and data analysis. Smart gas sensors can transmit data wirelessly, allowing for remote monitoring and alerts.
- Artificial Intelligence (AI) and Machine Learning (ML): AI and ML algorithms can be used to improve the performance of gas sensors by analyzing patterns in sensor data and predicting gas concentrations. This can lead to more accurate and reliable gas detection.
- Miniaturization and Wearable Gas Sensors:
- Advances in microelectronics and packaging technologies have enabled the miniaturization of gas sensors. Wearable gas sensors can be integrated into personal protective equipment (PPE) or clothing, providing real-time monitoring of harmful gases in the environment.
- Enhanced Selectivity and Sensitivity:
- Researchers are developing new materials and coating technologies to improve the selectivity and sensitivity of gas sensors. For example, the use of polymer coatings can enhance the selectivity of MOS sensors towards specific gases.
Applications of Gas Sensors
Gas sensors have a wide range of applications across various industries, including:
- Industrial Safety:
- Gas sensors are crucial for monitoring harmful gases in industrial settings, such as chemical plants, refineries, and mines. They help prevent accidents and ensure worker safety.
- Environmental Monitoring:
- Gas sensors are used to monitor air quality and detect pollutants such as NO2, SO2, and VOCs. This information is essential for environmental management and policy-making.
- Healthcare:
- Gas sensors have applications in healthcare, such as monitoring exhaled breath for medical diagnosis (e.g., detecting CO2 and O2 levels in respiratory patients) and monitoring anesthesia levels during surgery.
- Agriculture:
- Gas sensors can be used to monitor the composition of greenhouse gases and detect pests and diseases in crops by analyzing volatile compounds emitted by plants.
- Automotive:
- Gas sensors are used in automotive applications to detect exhaust gases (e.g., CO, NOx) and ensure compliance with emission standards. They also play a role in fuel efficiency and engine performance monitoring.
- Consumer Electronics:
- Gas sensors are increasingly being integrated into consumer electronics, such as smart homes and wearable devices, for monitoring indoor air quality and detecting harmful gases.
Future Trends and Challenges
The future of gas sensors is promising, with continued advancements in technology and materials science driving innovation. However, several challenges remain:
- Cost:
- Reducing the cost of gas sensors while maintaining high performance is a significant challenge. This is particularly important for widespread adoption in consumer electronics and emerging markets.
- Selectivity and Sensitivity:
- Improving the selectivity and sensitivity of gas sensors, especially in complex environments with multiple gases present, is ongoing research.
- Integration and Interoperability:
- Ensuring that gas sensors can seamlessly integrate with existing systems and platforms, such as IoT networks and smart city infrastructures, is crucial for widespread adoption.
- Standardization and Regulation:
- Developing international standards and regulations for gas sensors will help ensure their reliability, accuracy, and safety.
Conclusion
Gas sensors have evolved significantly over the past few decades, driven by advancements in technology and materials science. Modern gas sensors offer enhanced sensitivity, selectivity, and reliability, enabling a wide range of applications across various industries. The future of gas sensors is promising, with continued innovation and research driving advancements in technology, materials, and integration. However, challenges such as cost, selectivity, and integration remain, requiring ongoing research and development efforts. As gas sensors become more sophisticated and widespread, they will play an increasingly important role in monitoring and detecting harmful gases, ensuring safety, and protecting the environment.
Gas sensors have emerged as pivotal devices in various industries, playing a crucial role in monitoring and detecting harmful or flammable gases. With the rapid advancements in technology, gas sensors have evolved significantly, offering enhanced sensitivity, selectivity, and reliability. This article delves into the advancements in gas sensor applications, exploring the latest innovations, technologies, and trends shaping the future of gas detection.
Historical Evolution of Gas Sensors
The history of gas sensors dates back to the early 19th century, with the development of the first chemical gas detection methods. However, it was not until the mid-20th century that significant progress was made in creating practical and reliable gas sensors. Early gas sensors relied on chemical reactions or changes in electrical conductivity to detect gases. These sensors were often bulky, had limited sensitivity, and required frequent maintenance.
Advancements in materials science, microelectronics, and nanotechnology have revolutionized gas sensors. Modern gas sensors are smaller, more accurate, and capable of detecting a wider range of gases at lower concentrations. The evolution of gas sensors can be broadly categorized into several generations, each characterized by significant technological leaps.
- First-Generation Gas Sensors: These sensors relied on chemical reactions to detect gases. Examples include catalytic bead sensors for detecting combustible gases and metal oxide semiconductor (MOS) sensors for detecting reducing gases.
- Second-Generation Gas Sensors: These sensors utilized electrochemical principles, offering improved sensitivity and selectivity. Electrochemical sensors are widely used for detecting toxic gases such as carbon monoxide (CO) and hydrogen sulfide (H2S).
- Third-Generation Gas Sensors: This generation introduced optical sensors, which utilize the absorption, reflection, or fluorescence of light to detect gases. Optical sensors are highly selective and can operate at high temperatures, making them suitable for harsh environments.
- Fourth-Generation Gas Sensors: The latest generation of gas sensors leverages nanotechnology and advanced materials such as graphene, carbon nanotubes, and metal-organic frameworks (MOFs). These sensors offer unprecedented sensitivity, selectivity, and stability, enabling the detection of trace gases at parts-per-million (ppm) or even parts-per-billion (ppb) levels.
Types of Gas Sensors
Gas sensors can be classified based on their operating principles, detection mechanisms, and target gases. Some of the most common types of gas sensors include:
- Metal Oxide Semiconductor (MOS) Sensors:
- Operating Principle: Changes in electrical conductivity due to gas adsorption on the sensor surface.
- Applications: Detection of reducing gases (e.g., CO, H2, NH3) and oxidizing gases (e.g., NO2, O3).
- Advantages: Low cost, simple manufacturing process, and wide detection range.
- Disadvantages: Limited selectivity and sensitivity to humidity and temperature changes.
- Electrochemical Sensors:
- Operating Principle: Electrochemical reactions between the target gas and an electrolyte, resulting in a measurable current.
- Applications: Detection of toxic gases (e.g., CO, H2S, SO2) and oxygen.
- Advantages: High sensitivity and selectivity, fast response time.
- Disadvantages: Limited lifetime due to electrolyte consumption, and sensitivity to temperature and humidity.
- Optical Sensors:
- Operating Principle: Absorption, reflection, or fluorescence of light by the target gas.
- Applications: Detection of a wide range of gases, including hydrocarbons, CO2, and volatile organic compounds (VOCs).
- Advantages: High selectivity, long lifetime, and operation at high temperatures.
- Disadvantages: High cost and complexity.
- Catalytic Bead Sensors:
- Operating Principle: Catalytic combustion of the target gas on a heated bead, resulting in a temperature change.
- Applications: Detection of combustible gases (e.g., methane, propane).
- Advantages: Simple design and low cost.
- Disadvantages: Limited selectivity and sensitivity to environmental conditions.
- Solid-State Sensors:
- Operating Principle: Changes in electrical properties (e.g., resistance, capacitance) due to gas adsorption.
- Applications: Detection of a variety of gases, including oxygen, nitrogen dioxide, and ammonia.
- Advantages: High stability and reliability, low power consumption.
- Disadvantages: Limited detection range and sensitivity.
Recent Advancements in Gas Sensor Technology
Recent advancements in gas sensor technology have focused on improving sensitivity, selectivity, stability, and reducing the size and cost of sensors. Some of the key developments include:
- Nanotechnology-Based Sensors:
- Graphene-Based Sensors: Graphene, a single layer of carbon atoms, exhibits exceptional electrical and mechanical properties. Graphene-based gas sensors offer high sensitivity and fast response times. Research has shown that graphene can detect gases such as NO2, NH3, and CO at ppm levels.
- Carbon Nanotubes (CNTs): CNTs have a high surface-to-volume ratio, making them ideal for gas sensing. CNT-based sensors can detect a wide range of gases, including VOCs, CO, and H2.
- Metal-Organic Frameworks (MOFs): MOFs are crystalline materials with high porosity and large surface areas. They can be tailored to selectively adsorb specific gases, making them ideal for highly selective gas sensing.
- Smart Gas Sensors:
- Integration with IoT: The integration of gas sensors with the Internet of Things (IoT) enables real-time monitoring and data analysis. Smart gas sensors can transmit data wirelessly, allowing for remote monitoring and alerts.
- Artificial Intelligence (AI) and Machine Learning (ML): AI and ML algorithms can be used to improve the performance of gas sensors by analyzing patterns in sensor data and predicting gas concentrations. This can lead to more accurate and reliable gas detection.
- Miniaturization and Wearable Gas Sensors:
- Advances in microelectronics and packaging technologies have enabled the miniaturization of gas sensors. Wearable gas sensors can be integrated into personal protective equipment (PPE) or clothing, providing real-time monitoring of harmful gases in the environment.
- Enhanced Selectivity and Sensitivity:
- Researchers are developing new materials and coating technologies to improve the selectivity and sensitivity of gas sensors. For example, the use of polymer coatings can enhance the selectivity of MOS sensors towards specific gases.
Applications of Gas Sensors
Gas sensors have a wide range of applications across various industries, including:
- Industrial Safety:
- Gas sensors are crucial for monitoring harmful gases in industrial settings, such as chemical plants, refineries, and mines. They help prevent accidents and ensure worker safety.
- Environmental Monitoring:
- Gas sensors are used to monitor air quality and detect pollutants such as NO2, SO2, and VOCs. This information is essential for environmental management and policy-making.
- Healthcare:
- Gas sensors have applications in healthcare, such as monitoring exhaled breath for medical diagnosis (e.g., detecting CO2 and O2 levels in respiratory patients) and monitoring anesthesia levels during surgery.
- Agriculture:
- Gas sensors can be used to monitor the composition of greenhouse gases and detect pests and diseases in crops by analyzing volatile compounds emitted by plants.
- Automotive:
- Gas sensors are used in automotive applications to detect exhaust gases (e.g., CO, NOx) and ensure compliance with emission standards. They also play a role in fuel efficiency and engine performance monitoring.
- Consumer Electronics:
- Gas sensors are increasingly being integrated into consumer electronics, such as smart homes and wearable devices, for monitoring indoor air quality and detecting harmful gases.
Future Trends and Challenges
The future of gas sensors is promising, with continued advancements in technology and materials science driving innovation. However, several challenges remain:
- Cost:
- Reducing the cost of gas sensors while maintaining high performance is a significant challenge. This is particularly important for widespread adoption in consumer electronics and emerging markets.
- Selectivity and Sensitivity:
- Improving the selectivity and sensitivity of gas sensors, especially in complex environments with multiple gases present, is ongoing research.
- Integration and Interoperability:
- Ensuring that gas sensors can seamlessly integrate with existing systems and platforms, such as IoT networks and smart city infrastructures, is crucial for widespread adoption.
- Standardization and Regulation:
- Developing international standards and regulations for gas sensors will help ensure their reliability, accuracy, and safety.
Conclusion
Gas sensors have evolved significantly over the past few decades, driven by advancements in technology and materials science. Modern gas sensors offer enhanced sensitivity, selectivity, and reliability, enabling a wide range of applications across various industries. The future of gas sensors is promising, with continued innovation and research driving advancements in technology, materials, and integration. However, challenges such as cost, selectivity, and integration remain, requiring ongoing research and development efforts. As gas sensors become more sophisticated and widespread, they will play an increasingly important role in monitoring and detecting harmful gases, ensuring safety, and protecting the environment.
Historical Evolution of Gas Sensors
The history of gas sensors dates back to the early 19th century, with the development of the first chemical gas detection methods. However, it was not until the mid-20th century that significant progress was made in creating practical and reliable gas sensors. Early gas sensors relied on chemical reactions or changes in electrical conductivity to detect gases. These sensors were often bulky, had limited sensitivity, and required frequent maintenance.
Advancements in materials science, microelectronics, and nanotechnology have revolutionized gas sensors. Modern gas sensors are smaller, more accurate, and capable of detecting a wider range of gases at lower concentrations. The evolution of gas sensors can be broadly categorized into several generations, each characterized by significant technological leaps.
- First-Generation Gas Sensors: These sensors relied on chemical reactions to detect gases. Examples include catalytic bead sensors for detecting combustible gases and metal oxide semiconductor (MOS) sensors for detecting reducing gases.
- Second-Generation Gas Sensors: These sensors utilized electrochemical principles, offering improved sensitivity and selectivity. Electrochemical sensors are widely used for detecting toxic gases such as carbon monoxide (CO) and hydrogen sulfide (H2S).
- Third-Generation Gas Sensors: This generation introduced optical sensors, which utilize the absorption, reflection, or fluorescence of light to detect gases. Optical sensors are highly selective and can operate at high temperatures, making them suitable for harsh environments.
- Fourth-Generation Gas Sensors: The latest generation of gas sensors leverages nanotechnology and advanced materials such as graphene, carbon nanotubes, and metal-organic frameworks (MOFs). These sensors offer unprecedented sensitivity, selectivity, and stability, enabling the detection of trace gases at parts-per-million (ppm) or even parts-per-billion (ppb) levels.
Types of Gas Sensors
Gas sensors can be classified based on their operating principles, detection mechanisms, and target gases. Some of the most common types of gas sensors include:
- Metal Oxide Semiconductor (MOS) Sensors:
- Operating Principle: Changes in electrical conductivity due to gas adsorption on the sensor surface.
- Applications: Detection of reducing gases (e.g., CO, H2, NH3) and oxidizing gases (e.g., NO2, O3).
- Advantages: Low cost, simple manufacturing process, and wide detection range.
- Disadvantages: Limited selectivity and sensitivity to humidity and temperature changes.
- Electrochemical Sensors:
- Operating Principle: Electrochemical reactions between the target gas and an electrolyte, resulting in a measurable current.
- Applications: Detection of toxic gases (e.g., CO, H2S, SO2) and oxygen.
- Advantages: High sensitivity and selectivity, fast response time.
- Disadvantages: Limited lifetime due to electrolyte consumption, and sensitivity to temperature and humidity.
- Optical Sensors:
- Operating Principle: Absorption, reflection, or fluorescence of light by the target gas.
- Applications: Detection of a wide range of gases, including hydrocarbons, CO2, and volatile organic compounds (VOCs).
- Advantages: High selectivity, long lifetime, and operation at high temperatures.
- Disadvantages: High cost and complexity.
- Catalytic Bead Sensors:
- Operating Principle: Catalytic combustion of the target gas on a heated bead, resulting in a temperature change.
- Applications: Detection of combustible gases (e.g., methane, propane).
- Advantages: Simple design and low cost.
- Disadvantages: Limited selectivity and sensitivity to environmental conditions.
- Solid-State Sensors:
- Operating Principle: Changes in electrical properties (e.g., resistance, capacitance) due to gas adsorption.
- Applications: Detection of a variety of gases, including oxygen, nitrogen dioxide, and ammonia.
- Advantages: High stability and reliability, low power consumption.
- Disadvantages: Limited detection range and sensitivity.
Recent Advancements in Gas Sensor Technology
Recent advancements in gas sensor technology have focused on improving sensitivity, selectivity, stability, and reducing the size and cost of sensors. Some of the key developments include:
- Nanotechnology-Based Sensors:
- Graphene-Based Sensors: Graphene, a single layer of carbon atoms, exhibits exceptional electrical and mechanical properties. Graphene-based gas sensors offer high sensitivity and fast response times. Research has shown that graphene can detect gases such as NO2, NH3, and CO at ppm levels.
- Carbon Nanotubes (CNTs): CNTs have a high surface-to-volume ratio, making them ideal for gas sensing. CNT-based sensors can detect a wide range of gases, including VOCs, CO, and H2.
- Metal-Organic Frameworks (MOFs): MOFs are crystalline materials with high porosity and large surface areas. They can be tailored to selectively adsorb specific gases, making them ideal for highly selective gas sensing.
- Smart Gas Sensors:
- Integration with IoT: The integration of gas sensors with the Internet of Things (IoT) enables real-time monitoring and data analysis. Smart gas sensors can transmit data wirelessly, allowing for remote monitoring and alerts.
- Artificial Intelligence (AI) and Machine Learning (ML): AI and ML algorithms can be used to improve the performance of gas sensors by analyzing patterns in sensor data and predicting gas concentrations. This can lead to more accurate and reliable gas detection.
- Miniaturization and Wearable Gas Sensors:
- Advances in microelectronics and packaging technologies have enabled the miniaturization of gas sensors. Wearable gas sensors can be integrated into personal protective equipment (PPE) or clothing, providing real-time monitoring of harmful gases in the environment.
- Enhanced Selectivity and Sensitivity:
- Researchers are developing new materials and coating technologies to improve the selectivity and sensitivity of gas sensors. For example, the use of polymer coatings can enhance the selectivity of MOS sensors towards specific gases.
Applications of Gas Sensors
Gas sensors have a wide range of applications across various industries, including:
- Industrial Safety:
- Gas sensors are crucial for monitoring harmful gases in industrial settings, such as chemical plants, refineries, and mines. They help prevent accidents and ensure worker safety.
- Environmental Monitoring:
- Gas sensors are used to monitor air quality and detect pollutants such as NO2, SO2, and VOCs. This information is essential for environmental management and policy-making.
- Healthcare:
- Gas sensors have applications in healthcare, such as monitoring exhaled breath for medical diagnosis (e.g., detecting CO2 and O2 levels in respiratory patients) and monitoring anesthesia levels during surgery.
- Agriculture:
- Gas sensors can be used to monitor the composition of greenhouse gases and detect pests and diseases in crops by analyzing volatile compounds emitted by plants.
- Automotive:
- Gas sensors are used in automotive applications to detect exhaust gases (e.g., CO, NOx) and ensure compliance with emission standards. They also play a role in fuel efficiency and engine performance monitoring.
- Consumer Electronics:
- Gas sensors are increasingly being integrated into consumer electronics, such as smart homes and wearable devices, for monitoring indoor air quality and detecting harmful gases.
Future Trends and Challenges
The future of gas sensors is promising, with continued advancements in technology and materials science driving innovation. However, several challenges remain:
- Cost:
- Reducing the cost of gas sensors while maintaining high performance is a significant challenge. This is particularly important for widespread adoption in consumer electronics and emerging markets.
- Selectivity and Sensitivity:
- Improving the selectivity and sensitivity of gas sensors, especially in complex environments with multiple gases present, is ongoing research.
- Integration and Interoperability:
- Ensuring that gas sensors can seamlessly integrate with existing systems and platforms, such as IoT networks and smart city infrastructures, is crucial for widespread adoption.
- Standardization and Regulation:
- Developing international standards and regulations for gas sensors will help ensure their reliability, accuracy, and safety.
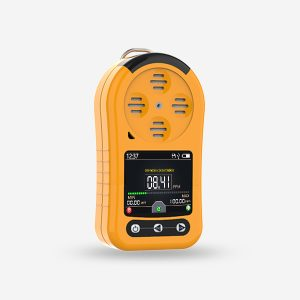
Gas sensors have evolved significantly over the past few decades, driven by advancements in technology and materials science. Modern gas sensors offer enhanced sensitivity, selectivity, and reliability, enabling a wide range of applications across various industries. The future of gas sensors is promising, with continued innovation and research driving advancements in technology, materials, and integration. However, challenges such as cost, selectivity, and integration remain, requiring ongoing research and development efforts. As gas sensors become more sophisticated and widespread, they will play an increasingly important role in monitoring and detecting harmful gases, ensuring safety, and protecting the environment.