Environmental monitoring is crucial for maintaining the health of ecosystems, ensuring public safety, and preventing disasters. Among the various tools used for this purpose, gas sensors play a pivotal role. These devices are designed to detect and measure the concentration of specific gases in the atmosphere, providing real-time data that can be analyzed to assess environmental conditions.
Recent advancements in gas sensor technology have significantly improved their accuracy, reliability, and versatility. This article explores the latest developments in gas sensor technology and their applications in environmental monitoring. We will discuss the types of gas sensors available, their operating principles, and the challenges faced in their deployment. Additionally, we will highlight the potential future directions in this field and the implications for environmental conservation.
Types of Gas Sensors
Gas sensors can be classified into several categories based on their operating principles and the gases they detect. The most common types include:
- Metal Oxide Semiconductor (MOS) SensorsMetal oxide semiconductor sensors are among the most widely used gas sensors due to their low cost, small size, and ease of use. They operate on the principle that the conductivity of a metal oxide film changes when it adsorbs a gas. This change in conductivity can be measured as a change in resistance, which is then converted into a concentration reading.MOS sensors are particularly effective in detecting combustible gases and certain toxic gases, such as carbon monoxide (CO) and sulfur dioxide (SO2). However, they can be sensitive to temperature and humidity changes, which can affect their accuracy.
- Chemiresistive SensorsChemiresistive sensors work similarly to MOS sensors but use different materials, such as polymers or carbon nanotubes, to detect gases. These materials change their resistance when exposed to specific gases, allowing for the measurement of gas concentrations.Chemiresistive sensors are often more selective than MOS sensors, making them useful for detecting a wider range of gases, including volatile organic compounds (VOCs) and ammonia (NH3). However, they can also be affected by temperature and humidity, and their long-term stability may be limited.
- Catalytic Bead SensorsCatalytic bead sensors use a catalytic material, such as platinum or palladium, to detect combustible gases. When a gas is adsorbed onto the catalytic bead, it undergoes a chemical reaction that produces heat. This heat can be measured and converted into a concentration reading.Catalytic bead sensors are highly sensitive to combustible gases, such as methane (CH4) and hydrogen (H2), and are often used in industrial safety applications. However, they can be sensitive to poisoning by certain gases, such as sulfur compounds, which can degrade their performance.
- Optical SensorsOptical sensors use light to detect gases. They can be based on absorption, fluorescence, or reflectance principles. In absorption-based sensors, the gas absorbs light at a specific wavelength, causing a decrease in the intensity of the transmitted light. This decrease can be measured and converted into a concentration reading.Fluorescence-based sensors use a material that fluoresces when excited by light of a specific wavelength. The presence of a gas can quench the fluorescence, causing a decrease in the intensity of the emitted light. Reflectance-based sensors measure the change in reflectance of a surface when a gas is adsorbed onto it.Optical sensors are highly selective and can detect a wide range of gases, including those that are difficult to detect using other methods. However, they can be expensive and require complex instrumentation.
- Electrochemical SensorsElectrochemical sensors measure the electrical current produced when a gas reacts with an electrolyte. This current is proportional to the concentration of the gas, allowing for accurate measurements.Electrochemical sensors are commonly used to detect oxygen (O2), carbon dioxide (CO2), and toxic gases, such as hydrogen sulfide (H2S) and chlorine (Cl2). They are highly sensitive and selective, but their lifetime is limited by the consumption of the electrolyte during the reaction.
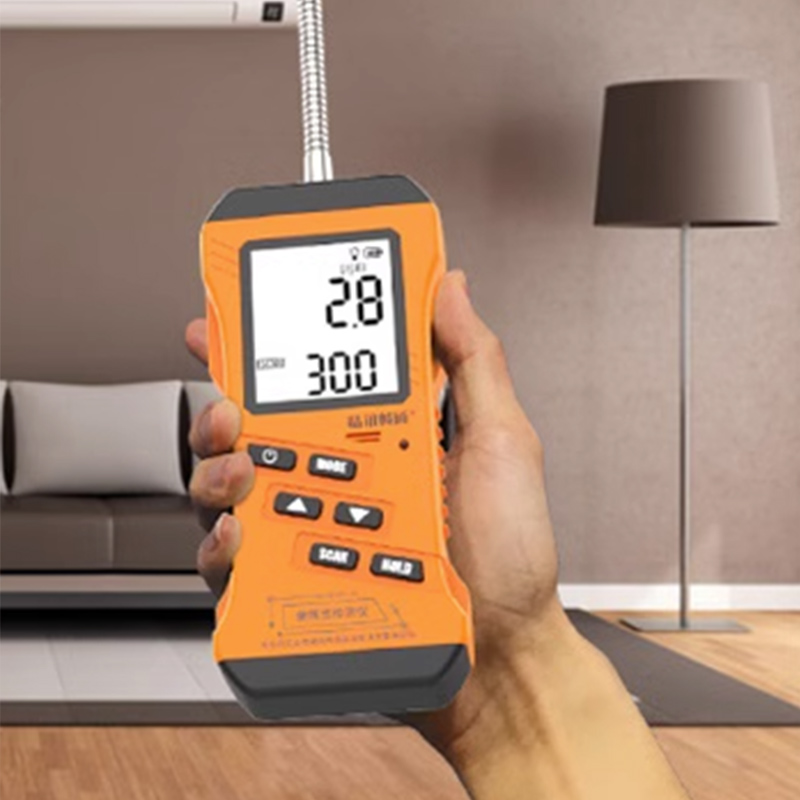
Operating Principles and Challenges
The operating principles of gas sensors vary depending on the type of sensor. However, most sensors rely on a physical or chemical interaction between the sensor material and the target gas. This interaction causes a change in a measurable property, such as resistance, temperature, or light intensity, which can be converted into a concentration reading.
Despite their advantages, gas sensors face several challenges in environmental monitoring. These include:
- SelectivityMany gases have similar chemical properties, making it difficult for sensors to distinguish between them. This can lead to false positives or negatives, reducing the accuracy of the measurements.
- SensitivitySensors must be able to detect gases at low concentrations, especially in environments where the gas levels are expected to be low. However, increasing sensitivity can also increase the risk of interference from other gases or environmental factors.
- StabilitySensor performance can degrade over time due to exposure to harsh environments, such as high temperatures, humidity, or corrosive gases. This can lead to drift in the measurements, requiring frequent calibration and maintenance.
- CostThe cost of gas sensors can vary widely depending on their type, complexity, and manufacturing process. High-end sensors, such as optical sensors, can be expensive and may not be feasible for widespread deployment.
- Data Integration and AnalysisThe data collected by gas sensors must be integrated and analyzed to provide meaningful insights. This requires sophisticated software and algorithms that can handle large datasets and extract useful information.
Applications in Environmental Monitoring
Gas sensors have a wide range of applications in environmental monitoring, including:
- Air Quality MonitoringGas sensors can be used to monitor air quality in urban and industrial areas. They can detect pollutants such as nitrogen oxides (NOx), sulfur dioxide (SO2), volatile organic compounds (VOCs), and particulate matter (PM). This information can be used to assess the health risks associated with air pollution and to develop effective mitigation strategies.
- Greenhouse Gas Emissions MonitoringGas sensors can be used to monitor emissions of greenhouse gases, such as carbon dioxide (CO2) and methane (CH4), from industrial sources, vehicles, and agricultural activities. This information can be used to track progress in reducing emissions and to develop policies to mitigate climate change.
- Indoor Air Quality MonitoringGas sensors can be used to monitor indoor air quality in buildings, such as offices, schools, and hospitals. They can detect pollutants such as formaldehyde, total volatile organic compounds (TVOCs), and carbon monoxide (CO). This information can be used to improve ventilation systems and reduce the risk of health problems associated with indoor air pollution.
- Emergency ResponseGas sensors can be used in emergency response situations, such as chemical spills or industrial accidents, to detect and monitor hazardous gases. This information can be used to evacuate affected areas and to develop response plans to mitigate the impact of the hazard.
- Agricultural MonitoringGas sensors can be used in agriculture to monitor the emissions of ammonia (NH3) and methane (CH4) from livestock operations and manure storage facilities. This information can be used to develop best management practices to reduce emissions and improve air quality in rural areas.
Future Directions and Implications
The field of gas sensor technology is rapidly evolving, with ongoing research and development efforts focused on improving sensor performance, reducing costs, and expanding their applications. Some of the potential future directions in this field include:
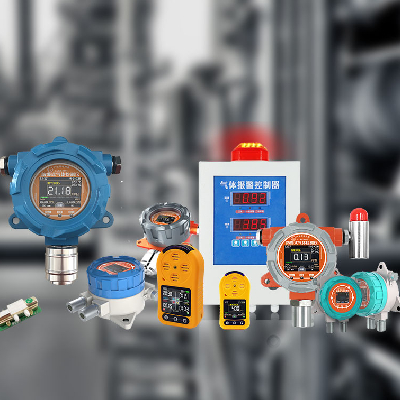
- Improved Selectivity and SensitivityResearchers are developing new materials and sensing mechanisms to improve the selectivity and sensitivity of gas sensors. This includes the use of nanomaterials, such as graphene and metal-organic frameworks (MOFs), which offer high surface areas and tunable pore sizes for enhanced gas adsorption and detection.
- Miniaturization and IntegrationAdvances in microelectronics and nanotechnology are enabling the miniaturization of gas sensors, making them smaller, lighter, and more energy-efficient. This is enabling the integration of gas sensors into smart devices, such as smartphones and wearable sensors, for real-time monitoring of air quality and personal exposure to pollutants.
- Wireless and Remote SensingThe development of wireless communication technologies is enabling the remote monitoring of gas concentrations over large areas. This includes the use of Internet of Things (IoT) devices, such as sensors equipped with GPS and radio frequency (RF) communication capabilities, for real-time data collection and analysis.
- Artificial Intelligence and Machine LearningThe integration of artificial intelligence (AI) and machine learning (ML) algorithms is enabling more sophisticated data analysis and decision-making based on gas sensor data. These algorithms can be used to identify patterns, predict trends, and develop early warning systems for potential environmental hazards.
The implications of these advancements in gas sensor technology for environmental monitoring are significant. They offer the potential for more accurate, reliable, and cost-effective monitoring of air quality and greenhouse gas emissions, enabling better decision-making and policy development to protect public health and the environment.
Conclusion
Gas sensors are essential tools for environmental monitoring, providing real-time data on air quality and greenhouse gas emissions. Recent advancements in gas sensor technology have significantly improved their performance, making them more accurate, reliable, and versatile. However, challenges such as selectivity, sensitivity, stability, cost, and data integration and analysis remain.